GLOSSARY - 4_0104910041GLOSSARY (cont.) - 4_0104910042GLOSSARY (cont.) - 4_0104910043GLOSSARY (cont.) - 4_0104910044GLOSSARY (cont.) - 4_0104910045GLOSSARY (cont.) - 4_0104910046GLOSSARY (cont.) - 4_0104910047GLOSSARY (cont.) - 4_0104910048GLOSSARY (cont.) - 4_0104910049GLOSSARY (cont.) - 4_0104910050GLOSSARY (cont.) - 4_0104910051GLOSSARY (cont.) - 4_0104910052GLOSSARY (cont.) - 4_0104910053GLOSSARY (cont.) - 4_0104910054GLOSSARY (cont.) - 4_0104910055GLOSSARY (cont.) - 4_0104910056GLOSSARY (cont.) - 4_0104910057GLOSSARY (cont.) - 4_0104910058GLOSSARY (cont.) - 4_0104910059GLOSSARY (cont.) - 4_0104910060GLOSSARY (cont.) - 4_0104910061CLASSIFICATION OF RIVER CROSSINGS AND ENCROACHMENTSDYNAMICS OF NATURAL RIVERS AND THEIR TRIBUTARIESFigure 1.1. Geometric properties of bridge crossingsFigure 1.2. Comparison of the 1884 and 1968 Mississippi River Channel near Commerce, Missouri.Historical Evidence of the Natural Instability of Fluvial SystemsHistorical Evidence of the Natural Instability of Fluvial Systems (cont.)Introduction to River Hydraulics and River ResponseFigure 1.3. Sinuosity vs. slope with constant dischargeEFFECTS OF HIGHWAY CONSTRUCTION ON RIVER SYSTEMSDelayed Response of Rivers to EncroachmentEFFECTS OF RIVER DEVELOPMENT ON HIGHWAY ENCROACHMENTSEFFECTS OF RIVER DEVELOPMENT ON HIGHWAY ENCROACHMENTS (cont.)TECHNICAL ASPECTSVariables Affecting River BehaviorFUTURE TECHNICAL TRENDSResearch NeedsOVERVIEW OF MANUAL CONTENTSChapter 3 - Fundamentals of Alluvial Channel FlowChapter 5 - River Morphology and River ResponseChapter 8 Data Need and Data SourcesTable 1.1. Commonly Used Engineering Terms in SI and English UnitsOPEN CHANNEL FLOWDefinitions - 4_0104910086THREE BASIC EQUATIONSFigure 2.1. A river reach as a control volumeConservation of MassFigure 2.2. The control volume for conservation of linear momentumConservation of Linear MomentumFigure 2.3. The streamtube as a control volumeConservation of EnergyConservation of Energy (cont.) - 4_0104910094Conservation of Energy (cont.) - 4_0104910095HYDROSTATICSFigure 2.4. Pressure distribution in steady uniform and in steady nonuniform flowFigure 2.6. Steady uniform flow in a unit width channelSTEADY UNIFORM FLOWFigure 2.7. Hydraulically smooth boundaryFigure 2.8. Hydraulically rough boundaryShear Stress, Velocity Distribution, and Average VelocityShear Stress, Velocity Distribution, and Average Velocity (cont.)Figure 2.10. Einstein's multiplication factor X in the logarithmic velocity equationsOther Velocity EquationsTable 2.1. Manning's Roughness Coefficients for Various BoundariesTable 2.1. Manning's Roughness Coefficients for Various Boundaries (cont.)Average Boundary Shear StressTable 2.2. Adjustment Factors for the Determination of n ValuesFigure 2.11. Control volume for steady uniform flowRelation Between Shear Stress and VelocityEnergy and Momentum Coefficients for RiversEnergy and Momentum Coefficients for Rivers (cont.) - 4_0104910113Figure 2.12. Energy and momentum coefficients for a unit width of riverEnergy and Momentum Coefficients for Rivers (cont.) - 4_0104910115Figure 2.14. Definition sketch for small amplitude wavesGravity WavesFigure 2.15. Sketch of positive and negative surgesHydraulic JumpFigure 2.16. Hydraulic jump characteristics as a function of the upstream Froude numberFigure 2.18. Roll waves or slug flowFigure 2.19. Transitions in open channel flowFigure 2.20. Specific energy diagramSpecific Discharge DiagramFigure 2.21. Changes in water surface resulting from an increase in bed elevationFigure 2.23. Change in water surface elevation resulting from a change in widthTransitions With Super Critical FlowsFigure 2.24. Flow characteristics over a drop structure (Chow 1959)Figure 2.25. Nappe profiles for supercritical flow (Ippen 1950)Transverse Velocity Distribution in BendsFigure 2.26. Schematic representation of transverse currents in a channel bedSubcritical Flow in BendsSupercritical Flow in BendsGRADUALLY VARIED FLOWClassification of Flow ProfilesFigure 2.28. Classification of water surface profilesTable 2.3. Characteristics of Water Surface ProfilesFigure 2.29. Examples of water surface profilesFigure 2.30. Definition sketch for the standard step method for computation of backwater curvesSTREAM GAGINGFigure 2.31. Gaging station well and shelters (from Buchanan and Somers 1968a)Figure 2.32. Typical float recording gaging station (from Buchanan and Somers 1968a)Figure 2.34. Stage vs. time hydrograph (Kennedy 1983)Figure 2.35. Definition sketch of computing area and discharge at a gaging stationFigure 2.36. Stage-discharge relation for Schoharie Creek, New York (Butch 2000)Figure 2.37. Stage-discharge relation for a sand channelBackwater Effects on Waterway OpeningsFigure 2.38. Three types of backwater effect associated with bridge crossingsFigure 2.39. Submergence of a superstructureFigure 2.40. Types of flow encountered (HDS 1, Bradley 1978)HYDRAULICS OF CULVERT FLOWROADWAY OVERTOPPINGFigure 2.41. Discharge coefficient for roadway overtoppingTable 2.4. Discharge measurement notesTable 2.5. Observed Velocity DataTable 2.6. Velocity Profile CalculationsShear Stress Analysis - 4_0104910157Table 2.7. Detailed Computation of Superelevation in BendsPROBLEM 4 Maximum Stream Constriction Without Causing Backwater (Neglecting Energy Losses) - 4_0104910159PROBLEM 5 Maximum Water Surface Elevation Upstream of a Grade Control Structure Without Backwater (Neglecting Energy Losses)Figure 2.43. Sketch of backwater curve over check damHow much backwater will the dam cause for a flow of 28.37 m3/s if the normal depth for this discharge is 1.52 m and the dam height is 1.22 m?SOLVED PROBLEMS OPEN CHANNEL FLOW (ENGLISH)Table 2.8. Discharge measurement notesTable 2.9. Observed Velocity DataTable 2.10. Velocity Profile CalculationsShear Stress Analysis - 4_0104910167Table 2.11. Detailed Computation of Superelevation in BendsPROBLEM 4 Maximum Stream Constriction Without Causing Backwater (Neglecting Energy Losses) - 4_0104910169PROBLEM 5 Maximum Elevation of a Grade Control Structure Without Backwater (Neglecting Energy Losses)Figure 2.44. Sketch of backwater curve over check damHow much backwater will the dam cause for a flow of 1000 cfs if the normal depth for this discharge is 5 ft and the dam height is 4.0 ft?How much backwater will the dam cause for a flow of 1000 cfs if the normal depth for this discharge is 5 ft and the dam height is 4.0 ft? (cont.)FUNDAMENTALS OF ALLUVIAL CHANNEL FLOWParticle ShapeTable 3.1. Sediment Grade Scale (Brown 1950)Fall Velocity - 4_0104910178Figure 3.1. Drag coefficient CD vs particle Reynolds number Rep for spheres and natural sediments with shape factors Sp equal to 0.3, 0.5, 0.7, and 0.9.Figure 3.2. Nominal diameter vs. fall velocity (Temperature = 24C)Sediment Size DistributionSquare-Surface SampleFigure 3.3. Definition sketches for size-frequency characteristics of sedimentsSediment Size Distribution (cont.)Specific WeightFLOW IN SANDBED CHANNELSFigure 3.4. Angle of repose of non-cohesive materialsFigure 3.5. Forms of bed roughness in sand channelsFigure 3.6. Relation between water surface and bed configurationFigure 3.7. Change in velocity with stream power for a sand with D50 = 0.19 mmPlane Bed Without Sediment MovementPlane Bed With MovemenRegime of Flow, Bed Configuration, and Froude NumberFigure 3.8. Relation between regime of flow and depth for bed material with a median size equal to or less than 0.35 mmRESISTANCE TO FLOW IN ALLUVIAL CHANNELSFigure 3.10. Relation of depth to discharge for Elkhorn River near Waterloo, NebraskaFigure 3.11. Apparent kinematic viscosity of water-bentonite dispersionsFigure 3.12. Variation of fall velocity of several sand mixtures with percent bentonite and temperatureSize GradationShape Factor for the Reach and Cross-SectionFigure 3.13. Relation between stream power, median fall diameter, and bed configuration and Manning's n valuesFigure 3.14. Change in Manning's n with discharge for Padma River in BangladeshAlluvial Processes and Resistance to Flow in Coarse Material StreamsResistance to FlowTable 3.3. Typical Sediment Size Distribution for Gravel-Bed StreamBEGINNING OF MOTIONTheory of Beginning of MotionTheory of Beginning of Motion (cont.) - 4_0104910208Theory of Beginning of Motion (cont.) - 4_0104910209Figure 3.15. Shields Diagram: dimensionless critical shear stressFigure 3.16. Shields' relation for beginning of motion (after Gessler 1971)Shields DiagramFigure 3.17. Comparison of critical shear stress as a function of grain diameteEquations for Flow and Sediment Variables for Beginning of MotionCritical Velocity for the Beginning of Bed Material MovementCritical Size for the Beginning of Bed Material MovementTable 3.4. Maximum Permissible Velocities Proposed by Fortier and Scobey (1926)Table 3.5. Nonscour Velocities for Soils (Modified from a report by Keown et al. 1977)Figure 3.19. Critical velocity as a function of stone sizeSEDIMENT DISCHARGE MEASUREMENTSuspended Sediment Discharge MeasurementFigure 3.21. Schematic sediment and velocity profilesFigure 3.22b. Suspended sediment sampler-DH48Figure 3.23. Gage height and suspended sediment concentration hydrograph, Colorado River near San Saba, Texas, May 1-6, 1952Total Sediment DischargeTable 3.6. Sand Bed Material Size DistributionTable 3.7. Sand Size Bed Material PropertiesTable 3.8. Gravel Bed Material Size DistributionTable 3.9. Gravel Bed Material PropertiesFigure 3.25. Size distribution curve for pebble count (1,000 mm = 1 m = 3.28 ft).PROBLEM 3 Resistance to Flow in Alluvial ChannelsManning's n in Cobble Bed Streams - 4_0104910232PROBLEM 4 Beginning of MotionCritical Velocity for Beginning of Bed Material MovementCritical Depth When the Bed Material Movement Would Stop - 4_0104910235PROBLEM 2 Angle of ReposeManning's n in Cobble Bed Streams - 4_0104910237Bed Material Movement Using Shields FigureCritical Size for Beginning of Bed Material MovementCritical Depth When the Bed Material Movement Would Stop - 4_0104910240SEDIMENT TRANSPORT - 4_0104910241DEFINITIONS - 4_0104910242GENERAL CONSIDERATIONSMode of Sediment TransportFigure 4.1. Classification of sediment transport in streams (rivers)Figure 4.2. Sediment transport capacity and supply curvesFigure 4.3. Schematic sediment and velocity profilesSUSPENDED BED SEDIMENT DISCHARGESUSPENDED BED SEDIMENT DISCHARGE (cont.)Figure 4.4. Graph of suspended sediment distribution (Rouse 1937).BED SEDIMENT DISCHARGEBED SEDIMENT DISCHARGE (cont.)Einstein's Method of Computing Bed Sediment DischargeEinstein's Method of Computing Bed Sediment Discharge (cont.)Figure 4.5. Einstein's φ* vs ψ * bed load function (Einstein 1950)Figure 4.6. Hiding factor (Einstein 1950)Figure 4.8. Pressure correction (Einstein 1950)Figure 4.9. Integral I1 in terms of E and Z (Einstein 1950)Figure 4.10. Integral I2 in terms of E and Z (Einstein 1950)Figure 4.11. Friction loss due to channel irregularities, as a function of sediment transportFigure 4.12. Comparison of the Meyer-Peter and Mller and Einstein methods for computing contact loadFigure 4.13. Relation of discharge of sands to mean velocity for six median sizes of bed sands, four depths of flow, and a water temperature of 60FFigure 4.14. Colby's correction curves for temperature and fine sedimentRelative Influence of VariablesFigure 4.15. Bed-material size effects on bed material transportFigure 4.17. Effect of kinematic viscosity (temperature) on bed material transportPOWER FUNCTION RELATIONSHIPSTable 4.1. Coefficient and Exponents of Equation 4.48Table 4.2. Range of Parameters Equation 4.48 Developed by Simons et al.Table 4.3. Coefficient and Exponents for Equation 4.50CONVERSION FACTORSAPPLICATION OF SELECTED SEDIMENT TRANSPORT EQUATIONSTable 4.4. Summary of Applicability of Selected Sediment Transport FormulasSEDIMENT TRANSPORTATION ANALYSIS PROCEDUREField MeasurementBasic Methods for Sediment Transport CalculationsSOLVED PROBLEMS FOR SEDIMENT TRANSPORT (SI)Table 4.5. Concentration vs. Elevation Above the BedProblem 3 Application of the Einstein Method to Calculate Total Bed-Material Discharge - 4_0104910279Problem 5 Calculation of Total Bed-Material Discharge Using the Basic Power Function RelationshipProblem 7 Calculate Total Bed-Material Discharge Using Yang's Sand Equation - 4_0104910281SOLVED PROBLEMS FOR SEDIMENT TRANSPORT (ENGLISH)Table 4.6. Concentration vs. Elevation Above the BedProblem 3 Application of the Einstein Method to Calculate Total Bed-Material Discharge - 4_0104910284Table 4.7. Bed Material Information for Sample ProblemFigure 4.20. Grain size distribution of bed materialTable 4.8. Hydraulic Calculations for Sample Problem 3. Application of the Einstein ProcedureTable 4.9. Bed-Material Load Calculations for Sample Problem by Applying the Einstein ProcedureTable 4.9. Bed-Material Load Calculations for Sample Problem by Applying the Einstein Procedure (cont.)Table 4.10. Bed Material Load Calculations for Sample Problem by Applying the Colby Method (Median Diameter)Table 4.11. Bed Material Discharge Calculations for Sample Problem by Applying the Colby (Individual Size Fraction)Figure 4.21. Comparison of the Einstein and Colby methodsProblem 6 Calculation of Total Bed-Material Discharge Using the Expanded Power Function RelationshipProblem 7 Calculate Total Bed-Material Discharge Using Yang's Sand Equation - 4_0104910294RIVER MORPHOLOGY AND RIVER RESPONSEFloodplain and Delta FormationsNickpoint Migration and HeadcuttingFigure 5.1. Changing slope at fan-head leading to fan-head trenchingVARIABILITY AND CHANGE IN ALLUVIAL RIVERSVARIABILITY AND CHANGE IN ALLUVIAL RIVERS (cont.)Figure 5.3. Relationship between flume slope and sinuosity (ratio between channel length and valley length) during flume experiments at constant water dischargeFigure 5.5. Variability of sinuosity between 1765 and 1915 for 24 Mississippi River reachesFigure 5.6. Mississippi River reaches between Cairo, Illinois and Old River, LouisianaDifferences Between ReachesFigure 5.8. River Nile between Qena and Cairo showing six reaches of steep valley slopeFigure 5.10. Plot of River Nile sinuosity and valley slope. Numbers represent reaches of Figure 5.9Classification of River ChannelsFigure 5.11. Stream properties for classification (after Brice and Blodgett 1978)Figure 5.12. Classification of river channels (after Culbertson et al. 1967)Figure 5.13. Channel classification showing relative stability and types of hazards encountered with each patternStraight River ChannelsStraight River Channels (cont.)Figure 5.15. Definition sketch for meandersFigure 5.16. Empirical relations for meander characteristics (Leopold et al. 1964)Figure 5.17. Types of multi-channel streamsFigure 5.18a,b. Slope-discharge relationship for braiding or meandering in sandbed streamsHydraulic Geometry of Alluvial ChannelsHydraulic Geometry of Alluvial Channels (cont.)Figure 5.19. Variation of discharge at a given river cross section and at points downstreamTable 5.2. At-A-Station and Downstream Hydraulic Geometry RelationshipsDominant Discharge in Alluvial RiversRiver Profiles and Bed MaterialGeneral River Response to ChangeFigure 5.21. Changes in channel slope in response to an increase in sediment load at Point C.Figure 5.22. Changes in channel slope in response to a dam at point CTable 5.4. Change of Variables Induced by Changes in Sediment Discharge, Size of Bed Sediment and Wash LoadTable 5.5. Qualitative Response of Alluvial ChannelsRiver Pattern Thresholds and ResponseTable 5.6. Chippewa River MorphologyFigure 5.23. Map of lower Chippewa River. The river is braided below Durand, Wisconsin.Figure 5.24. Leopold and Wolman's (1957) relation between channel patterns, channel gradient, and bankfull discharge.River Pattern Thresholds and Response (cont.)MODELING OF RIVER SYSTEMSPhysical ModelingPhysical Modeling (cont.)Table 5.7. Scale Ratios for Similitude.Computer ModelingComputer Modeling (cont.)BRI-STARS MODEL (The Bridge Stream Tube Model for Alluvial River Simulation)FLUVIAL-12Two-Dimensional Sediment Transport ModelsHIGHWAY PROBLEMS RELATED TO GRADATION CHANGESChanges Due to Human ActivitiesNatural CausesResulting Problems at Highway CrossingsTable 5.8. Channel Response to Changes in Watershed and River ConditionBank Stability - 4_0104910347Stability Problems Associated With Channel RelocationFigure 5.26. Median bank erosion rate in relation to channel width for different types of streamsFigure 5.27. Encroachment on a meandering riverStability Problems Associated With Channel Relocation (cont.) - 4_0104910351Stability Problems Associated With Channel Relocation (cont.) - 4_0104910352Assessment of Stability for Relocated StreamsEstimation of Future Channel Stability and BehaviorFigure 5.28. Erosion index in relation to sinuosity (Brice 1984)Figure 5.29. Modes of meander loop development.Assessment Of DegradationAdvances in Predicting Meander MigrationFigure 5.30. Domains of meander behavior Analysis OptionsFigure 5.31. Sinuous point bar streamFigure 5.32. River channels (After Petersen 1986)PROBLEM 3 Channel Response to Changes in Watershed ConditionsFigure 5.33. Meandering river sketch (after Petersen 1986)PROBLEM 5 At-A-Station and Downstream Hydraulic Geometry Relationships (SI)PROBLEM 5 At-A-Station and Downstream Hydraulic Geometry Relationships (SI) (cont.)PROBLEM 6 At-A-Station and Downstream Hydraulic Geometry Relationships (English)PROBLEM 7 Downstream Sediment Size DistributionTable 5.9. Sediment Size Distribution in the St. Lawrence SeawayPROBLEM 9 Scale Ratios for Physical Models (English)RIVER STABILIZATION AND BANK PROTECTIONTable 6.1. Factors Affecting Erosion of River BanksBed and Bank MaterialFigure 6.1. Typical bank failure surfaces (a) noncohesive, (b) cohesive, (c) compositePiping of River BanksRIVER TRAINING AND STABILIZATIONCountermeasures for Channel InstabilityCountermeasure Design GuidelinesProtection of Training WorksFLOW CONTROL STRUCTURESFigure 6.2. Placement of flow control structures relative to channel banks, crossing, and floodplainFigure 6.3. Perspective of hard point with section detailFigure 6.4. Retard.Dikes (Floodplain)Figure 6.6. Pile dikes (retards would be similar)Figure 6.8. Typical jetty-field layoutFigure 6.9. Steel jacksFigure 6.10. Typical guidebankFigure 6.11. Definition sketch for a vertical dropFigure 6.12. Flow and scour patterns at a sloping sillFigure 6.13. Diagram for riprap stability conditions.Stability Factor Design MethodsSimplified Design Aid For Side Slope RiprapFigure 6.14. Stability numbers for a 1.5 stability factor for horizontal flow along a side slopeU.S. Army Corps of Engineers Design EquationU.S. Army Corps of Engineers Design Equation (cont.) - 4_0104910396U.S. Army Corps of Engineers Design Equation (cont.) - 4_0104910397Figure 6.15. Suggested gradation for riprapRiprap Gradation and ThicknessRiprap PlacementFigure 6.16. Tie-in trench to prevent riprap blanket from unravelingWindrow RevetmentFigure 6.18. Windrow revetment, definition sketchRiprap Failure ModesFigure 6.19. Riprap failure modelsTable 6.3. Causes of Riprap Failure and SolutionsBioengineering CountermeasuresFigure 6.20. Rock and wire mattressGabions - 4_0104910409Figure 6.21. Typical sand-cement bag revetmentigure 6.22 Articulated concrete block systemSoil CementFigure 6.24. Typical soil-cement bank protectionOVERTOPPING FLOW ON EMBANKMENTSFigure 6.25. Hydraulic flow zones on an embankment during overtopping flowFigure 6.26. Definition sketch of variables involved in overtopping flowFigure 6.27. Definition sketch for application of the momentum equation for embankment overtopping flowFigure 6.28. Typical embankment erosion pattern with submerged flowFigure 6.29. Typical embankment erosion pattern with free flowErosion Protection in Overtopping FlowFigure 6.30. Permissible velocities of various protection materials as a function of flow durationFigure 6.31. Full-scale test of an embankment overtopping protection system under steep-slope, high-velocity flow conditionsENVIRONMENTAL CONSIDERATIONS - 4_0104910423Effects of ChannelizationSOLVED PROBLEMS FOR STABILITY OF RIPRAP (SI)Figure. 6.32. Definition sketch for riprap on a channel bedPROBLEM 3 Stability Factors for Riprap Design - 4_0104910427PROBLEM 3 Stability Factors for Riprap Design (cont.)Figure 6.33. Stability factors for various rock sizes on a side slopePROBLEM 4 Riprap Design on an AbutmentSOLVED PROBLEMS FOR FILTER DESIGN (SI)Table 6.5. Sizes of MaterialsPROBLEM 2 Filter Design - 4_0104910433Figure 6.35. Gradations of filter blanket for Problem 2 (after Anderson et al. 1968)Figure. 6.36. Definition sketch for riprap on a channel bedPROBLEM 2 Riprap Design on Embankment SlopesPROBLEM 3 Stability Factors for Riprap Design - 4_0104910437Figure 6.37. Stability factors for various rock sizes on a side slopeFigure 6.38. Safety factors for various side slopesSOLVED PROBLEMS FOR FILTER DESIGN (ENGLISH)PROBLEM 1 Filter DesignTable 6.7. Sizes of MaterialsPROBLEM 2 Filter Design - 4_0104910443Figure 6.39. Gradations of filter blanket for Problem 2SCOUR AT BRIDGESBridge Scour Evaluation ProgramFigure 7.1. Flow chart for scour and stream stability analysis and countermeasuresTOTAL SCOURFigure 7.2. Local scour depth at a pier as a function of timeLONG-TERM BED ELEVATION CHANGESGENERAL SCOURContraction ScourCritical VelocityLive-Bed Contraction ScourClear-water Contraction ScourLOCAL SCOUR AT PIERSComparison of Pier Scour EquationsFigure 7.3 Comparison of scour formulas for variable depth ratios (y/a)Figure 7.5. Comparison of pier scour equations with field measurementsFHWA HEC-18 EquationFigure 7.6. Common pier shapesCorrection Factor for Bed Material SizePier Scour in Cohesive Bed MaterialPier Scour for Other Pier Geometry, Flow Conditions, and DebrisFigure 7.7. Topwidth of scour hole (HEC-18)Figure 7.8. Schematic representation of abutment scourFigure 7.9. Comparison of laboratory flow characteristics to field flow conditionsFigure 7.10. Orientation of embankment angle θ to the flowFigure 7.11. Abutment shapeFroehlich's Live-Bed Scour EquationSCOUR PROBLEMSDATA NEEDS AND DATA SOURCESAerial and Other PhotographsClimatologic DataHydrologic Data - 4_0104910476CHECKLIST OF DATA NEEDSTable 8.1. Checklist of Data NeedsTable 8.1. Checklist of Data Needs (cont.)Table 8.2. List of Data SourcesTable 8.2. List of Data Sources (cont.) - 4_0104910481Table 8.2. List of Data Sources (cont.) - 4_0104910482CIVIL ENGINEERING DATABASEGeoRefDESIGN CONSIDERATIONS FOR HIGHWAY ENCROACHMENTS AND RIVER CROSSINGSLocation of the Crossing or EncroachmentHydrologic Data - 4_0104910487Flow AlignmentSite Selection - 4_0104910489PROCEDURE FOR EVALUATION AND DESIGN OF RIVER CROSSINGS AND ENCROACHMENTSFigure 9.1. Three-level analysis procedure for river engineering studiesCONCEPTUAL EXAMPLES OF RIVER ENCROACHMENTSCONCEPTUAL EXAMPLES OF RIVER ENCROACHMENTS (cont.) - 4_0104910493Table 9.1. River Response to Highway Encroachments and to River DevelopmentCONCEPTUAL EXAMPLES OF RIVER ENCROACHMENTS (Cont.) - 4_0104910495Table 9.1. River Response to Highway Encroachments and to River Development Table 9.1. River Response to Highway Encroachments and to River Development (continued) - 4_0104910497Table 9.1. River Response to Highway Encroachments and to River Development (continued) - 4_0104910498Table 9.1. River Response to Highway Encroachments and to River Development (continued) - 4_0104910499Table 9.1. River Response to Highway Encroachments and to River Development (continued) - 4_0104910500Table 9.1. River Response to Highway Encroachments and to River Development (continued) - 4_0104910501Table 9.1. River Response to Highway Encroachments and to River Development (continued) - 4_0104910502Table 9.1. River Response to Highway Encroachments and to River Development (continued) - 4_0104910503PRACTICAL EXAMPLES OF RIVER ENCROACHMENTSFigure 9.2. Cimarron River, east of Okeene, Oklahoma (Example 1)Figure 9.3. Arkansas River, north of Bixby, Oklahoma (Example 2)Figure 9.4. Washita River, north of Maysville, Oklahoma (Example 3)Figure 9.5. Beaver River, north of Laverne, Oklahoma (Example 4)Figure 9.6. Powder River, 40 miles east of Buffalo, Wyoming (Example 5)Figure 9.7. North Platte River near Guernsey, Wyoming (Example 6)Figure 9.8. Coal Creek, tributary of Powder River, Wyoming (Example 7)Figure 9.9b. Channel modifications to South Fork of Deer River at U.S. Highway 51 near Halls, Tennessee (Example 8)Figure 9.9c. Elevation sketch of U.S. Highway 51 Bridge (Example 8)Elk Creek at SR-15 Near Jackson, Nebraska (Example 9)Figure 9.10. Stage trends at Sioux City, Iowa, on the Missouri River (Example 9)Figure 9.11. Deflector arrangement and alignment problem on I-90 Bridge across Big Elk Creek near Piedmont, North Dakota (Example 10)Figure 9.12. Plan sketch of channel relocation, Outlet Creek (Example 11)Figure 9.13. Plan sketch of Nojoqui Creek channel relocation (Example 12)Figure 9.14. Plan sketch of Turkey Creek channel relocation (Example 13)Figure 9.15. Case study of sand and gravel mining (Example 14)Nowood River and Ten Sleep Creek Confluence, Wyoming (Example 15)Figure 9.16. Nowood River near Ten Sleep, Wyoming (Example 15)Figure 9.17. Middle Fork Powder River at Kaycee, Wyoming (Example 16)OVERVIEW EXAMPLES OF DESIGN F0R HIGHWAYS IN THE RIVER ENVIRONMENTLevel 1 - Reconnaissance and Geomorphic AnalysisFigure 10.2. Cross sectional area versus flow depth relationFigure 10.3. Wetted perimeter versus flow depth relationLevel 2 - Quantitative Engineering Analysis - 4_0104910529Figure 10.5. Gumbel's method of frequency analysis. Annual floods on Bijou Creek near Wiggins, ColoradoFigure 10.7. Proposed second alternativeFigure 10.9. Proposed fifth alternativeLevel 2 - Quantitative Engineering Analysis - 4_0104910533Table 10.2. Summary of Hydraulic DesignsTable 10.3. Design of Riprap SizesFigure 10.10. The sketch of proposed riprap designDesign for Alternatives 4 and 5Table 10.4. Bank Migration for Alternative 4.Table 10.7. Size and Filter Design for Riprap at Spur Noses and Spur ShanksFigure 10.11. Sketch of spur designOVERVIEW EXAMPLE 2 - RILLITO RIVERFigure 10.12. Rillito River system vicinity mapLevel 1 - Qualitative Geomorphic AnalysisLevel 1 - Qualitative Geomorphic Analysis (cont.)Figure 10.13. Slope-discharge relationship for Rillito RiverFigure 10.14. Sketch of Craycroft Road crossingLevel 2 - Engineering Geomorphic AnalysisFigure 10.15. Sabino Canyon Road crossing siteTable 10.8. Rillito River Near Tucson, Arizona Log-Pearson Type III Frequency Analysis by USGSFigure 10.17. Log-normal frequency analysis for Rillito River near TucsonFigure 10.19. 100-year flood design hydrographsFigure 10.21. Rillito-Pantano-Tanque Verde bed sediment distributionRiparian VegetationEngineering GeomorphologyTable 10.9. Equilibrium Slope Calculations, Dominant DischargeResults of AnalysisFigure 10.22. Bed elevation change of Rillito-Tanque Verde System (Tanque Verde flooding).Figure 10.24. Alternative I for Craycroft Road Bridge crossingFigure 10.25. Alternative II for Craycroft Road Bridge crossingTable 10.10. Low Chord and Total Scour Requirements at Craycroft RoadFigure 10.26. Alternative III for Craycroft Road Bridge crossingBRI-STARS SEDIMENT TRANSPORT MODELING EXAMPLEFigure 10.27. General plan view of Sabino Canyon Road crossing for alternative II, III, and IV (II Shown).Figure 10.28. Cross section of proposed bridgeLevel 2 - Hydraulic Engineering AnalysisFigure 10.29. Equal conveyance tubes of approach sectionFigure 10.31. Velocity distribution at bridge crossingFigure 10.32. Plot of total scour for example problemFigure 10.33. Revised plot of total scour for example problemFigure 10.34. Three-day hydrograph for BRI-STARS analysisFigure 10.36 Comparison cross-sections from BRI-STARSFigure 10.37. BRI-STARS model profiles for steady-state runREFERENCES - 4_0104910573REFERENCES (cont.) - 4_0104910574REFERENCES (cont.) - 4_0104910575REFERENCES (cont.) - 4_0104910576REFERENCES (cont.) - 4_0104910577REFERENCES (cont.) - 4_0104910578REFERENCES (cont.) - 4_0104910579REFERENCES (cont.) - 4_0104910580REFERENCES (cont.) - 4_0104910581REFERENCES (cont.) - 4_0104910582REFERENCES (cont.) - 4_0104910583REFERENCES (cont.) - 4_0104910584REFERENCES (cont.) - 4_0104910585REFERENCES (cont.) - 4_0104910586REFERENCES (cont.) - 4_0104910587REFERENCES (cont.) - 4_0104910588REFERENCES (cont.) - 4_0104910589REFERENCES (cont.) - 4_0104910590REFERENCES (cont.) - 4_0104910591REFERENCES (cont.) - 4_0104910592REFERENCES (cont.) - 4_0104910593REFERENCES (cont.) - 4_0104910594REFERENCES (cont.) - 4_0104910595REFERENCES (cont.) - 4_0104910596REFERENCES (cont.) - 4_0104910597REFERENCES (cont.) - 4_0104910598REFERENCES (cont.) - 4_0104910599REFERENCES (cont.) - 4_0104910600APPENDIX A. Metric System, Conversion Factors, and Water PropertiesTable A.1. Overview of SI Units.Table A.3. Derived Units With Special NamesTable A.4. Useful Conversion FactorsTable A.4. Useful Conversion Factors (cont.)Table A6. Physical Properties of Water at Atmospheric Pressure in SI UnitsTable A7. Physical Properties of Water at Atmospheric Pressure in English UnitsTable A8. Sediment Particles Grade ScaleTable A9. Common Equivalent Hydraulic UnitsAPPENDIX B. Analysis of Selected Sediment Transport RelationshipsEVALUATION OF SELECTED SEDIMENT TRANSPORT EQUATIONSTable B.1a. Field DataTable B.1b. Laboratory DataTable B3. Summary of Ten Sediment Transport RelationsAnalysis of Sediment Transport RelationsFigure B.1. Predicted versus measured total bed-material transport considering four classifications of size of bed materialsFigure B.1. Predicted versus measured total bed-material transport considering four classifications of size of bed materials (cont.) - 4_0104910618Figure B.1. Predicted versus measured total bed-material transport considering four classifications of size of bed materials (cont.) - 4_0104910619Figure B.1. Predicted versus measured total bed-material transport considering four classifications of size of bed materials (cont.) - 4_0104910620Figure B.2. Comparison Cppm computed and measured for three river setsFigure B.2. Comparison Cppm computed and measured for three river sets (cont.) - 4_0104910622Figure B.2. Comparison Cppm computed and measured for three river sets (cont.) - 4_0104910623Medium to very coarse sand-bed rivers (0.250 mm < D50 < 2.00 mm)Table B.4. Summary of Applicability of Selected Sediment Transport RelationsTable B.5. Coefficient and Exponents of Equation B.3Figure B.3. Relation of unit sediment discharge qs from measured and computed results using Equation B.5 for 2503 sets of field dataTable B.7. Range of Data Utilized by Posada to Develop Equation B.5LAURSEN AND MODIFIED LAURSEN EQUATIONSFigure B.4. Comparison of measured qs and computed qs using Posada and Kodoatie equations for various river-bed materials, data from Group 1Figure B.5. Comparison of measured qs and computed qs using Posada and Kodoatie equations for various river-bed materials, data from Group 2Figure B.6. Modifications of Laursen's (1958) GraphFigure B.7. Plotting V*/ωi and f(V*/ωi) for data from Group 1 with Laursen's EquationTable B.11. Value of "a" in Equation B.10 for Various Sizes of Bed Material.Figure B.8. Proposed graph by Kodoatie using Equation B.10Medium to Very Coarse Sand-Bed RiversVery Fine to Fine Sand-Bed RiversRANGE OF DATA FOR VALIDATIONBed Material SizeRiver SizeAPPENDIX C. IndexAPPENDIX C. Index (cont.) - 4_0104910642APPENDIX C. Index (cont.) - 4_0104910643APPENDIX C. Index (cont.) - 4_0104910644APPENDIX C. Index (cont.) - 4_0104910645APPENDIX C. Index (cont.) - 4_01049106464_010491
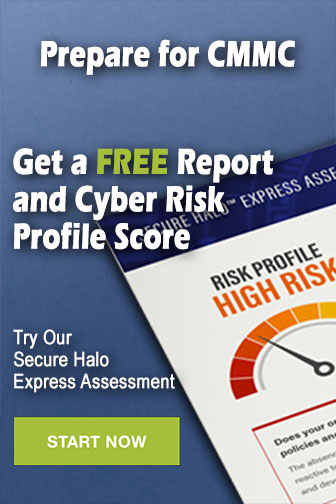